Reference: Cotella, E. M., Morano, R. L., Wulsin, A. C., Martelle, S. M., Lemen, P., Fitzgerald, M., Packard, B. A., Moloney, R. D., & Herman, J. P. (2020). Lasting Impact of Chronic Adolescent Stress and Glucocorticoid Receptor Selective Modulation in Male and Female Rats. Psychoneuroendocrinology, 112, 104490.
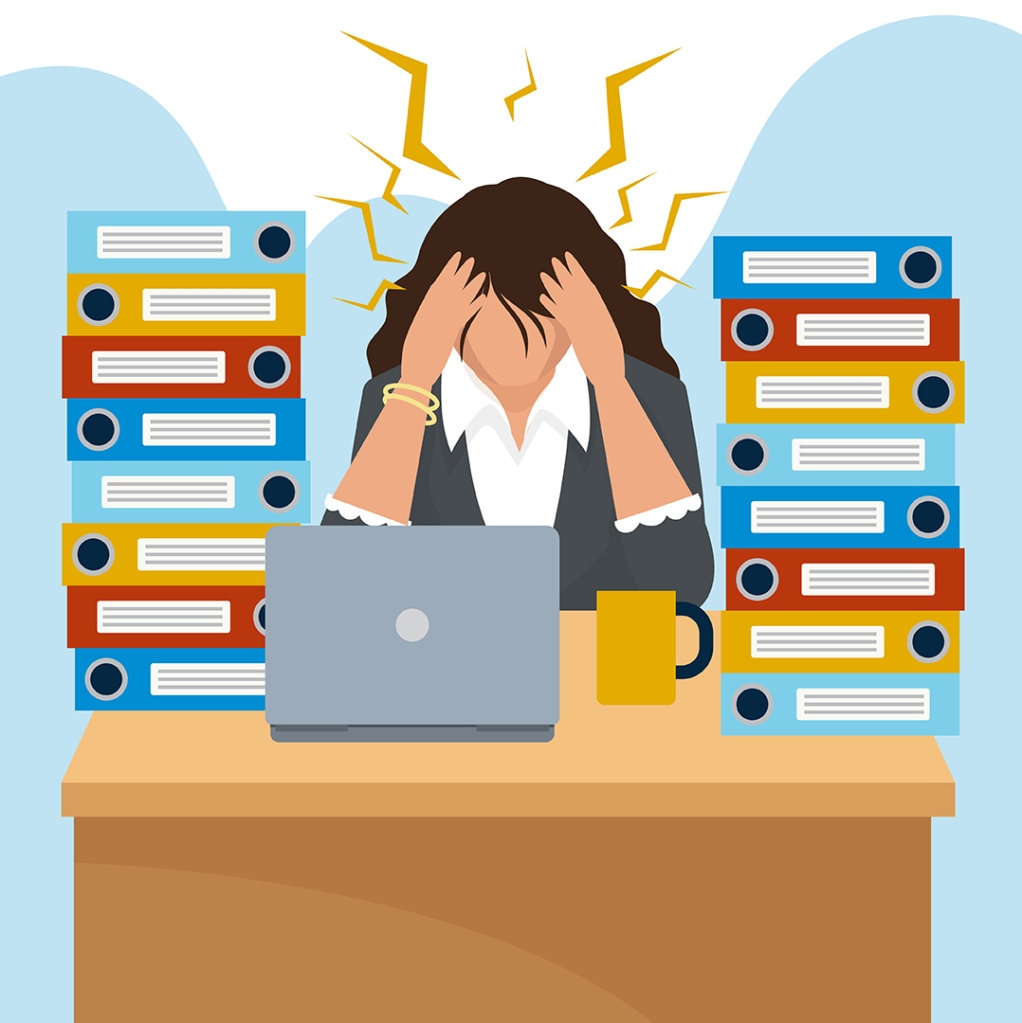
Coping with stress comes in many different shapes and forms. Some choose to escape with a book or TV series, some turn to their favorite hobby or try to burn off some steam with exercise, while others may choose to relax with some self-care or a good nap. While daily stressors are inescapable and often out of our control, how we cope with them can drastically affect our mental health. Identifying what separates those who maintain healthy responses to stress from others who are unable to manage adaptive coping strategies is imperative to understanding how stress shapes the brain. Additionally, maladaptive coping strategies are core features of conditions such as depression, PTSD, and substance abuse, so investigating how stress alters behavior can also promote our understanding of mental health disorders. Because adolescent stress is often connected to later mental health diagnoses, researchers at University of Cincinnati investigated how chronic adolescent stress may impact stress coping mechanisms later in adulthood.
Glucocorticoid Receptors’ Role in Stress
The hypothalamic-pituitary-adrenal (HPA) axis (shown in the figure below) is the main stress-responsive system which connects the brain and body. To put it simply, stressful stimuli trigger two brain areas — the hypothalamus and anterior pituitary — to release neurohormones, which in turn cause release of the stress hormone cortisol (or corticosterone in rodents) from the adrenal glands. Cortisol then enters the bloodstream and triggers aspects of “fight or flight” response, which help our bodies perform optimally when under duress. Importantly, cortisol exerts these actions by binding glucocorticoid receptors (GRs), which are widely expressed throughout the body (1). This binding of cortisol to GRs also prevents further release of cortisol — an action called negative feedback. This negative feedback function is essential for controlling the release of cortisol and ensuring we return to physiological baseline conditions when stress is over (2). Dysregulated HPA axis function can result from chronic stress and is also observed in conditions such as depression, bipolar disorder, and schizophrenia (3). Because adolescence is a critical developmental window for proper HPA axis function, and because less is known about the consequences of stress at this time, researchers in the Herman Lab aimed to address how chronic adolescent stress would alter stress coping behavior and HPA axis activity in adulthood.
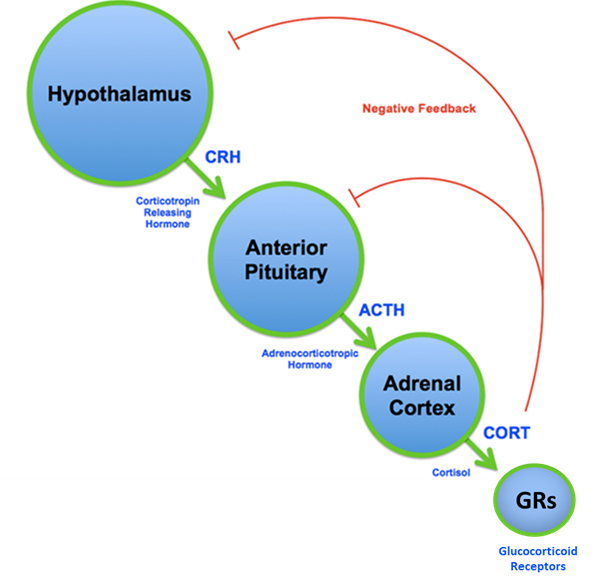
Chronic Variable Stress During Adolescence
In adolescence (when rats are around 46 days old), rats were subjected to 14 days of chronic variable stress during which animals undergo a variety of unpredictable stressful events, such as wet bedding, cold temperatures, and physical restraint twice daily for 30 minutes to an hour. Injections of the glucocorticoid receptor (GR) modulator CORT108297 were administered daily at the time of stress to interrupt GR signaling. Separate groups of animals were given control injections not containing the GR modulator to account for any stress that may be caused by the injection itself (see experimental groups below). By using CORT108297 to bind GRs and dampen GR signaling at the time of stress, researchers could study how GR signaling contributed to development of the long-term consequences of adolescent stress. Following chronic stress, the rats were given a 5-week rest period to assess coping strategies in adulthood and ascertain how blocking GRs would impact these outcomes.
No stress and control injections | Stress and control injections |
No stress and CORT108297 injections | Stress and CORT108297 injections |
Measuring Coping in Adulthood
Nine weeks following adolescent stress, the rats underwent the forced swim test to measure coping style in adulthood. During the forced swim test, the rat is placed in an inescapable container of water (see figure below) and behavior is scored and divided into two categories: active (like swimming and escape attempts) and passive (like time spent immobile). When placed in the water, survival instinct kicks into high gear and animals engage in active behavioral strategies to remain afloat. Eventually, however, animals “give up” and become immobile (don’t worry, they float!), which is viewed as passive coping. Because passive coping strategies in humans can be considered maladaptive and characteristic of mental health disorders such as depression, the forced swim test can be used to measure depressive-like symptoms in rodents (4). In fact, antidepressants have been shown to increase time spent engaging in active behaviors and decrease time immobile during forced swim (4,5). This study used the forced swim test to examine how stress would alter adult coping strategies, and if blocking GRs during adolescence would impact these measures.
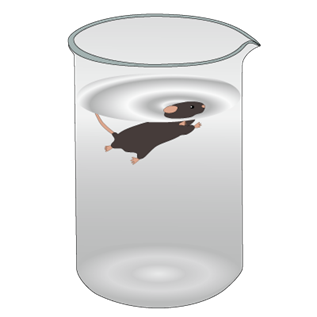
Sex-Specific Alterations in Coping Strategy
Interestingly, results varied based on the sex of the animal (see figure below). In male rats, adolescent stress did not alter total time spent immobile during forced swim (A). However, when the 10-minute test was broken down into 1-minute bins and analyzed separately, researchers found that stress increased time spent immobile in the last minute of the test (B). Unexpectedly, females displayed a robust shift in coping strategy following adolescent stress. Total immobility time during forced swim for females was significantly higher following adolescent stress (C), indicating a history of stress may induce a lasting shift towards passive coping strategies in females.
When broken down into 1-minute time bins, the GR modulator was shown to attenuate this stress-induced increase in immobility in the last minute of the test in males and females (B,D). This suggests GR modulation may mildly rescue adolescent stress-induced deficits. Importantly, both male and female controls who had received the GR modulator during adolescence with no history of stress displayed high immobility times in the last few minutes of the forced swim test (B,D). Independent of adolescent stress exposure, this finding could signify the importance of adolescent HPA axis activity on proper development of coping strategies.
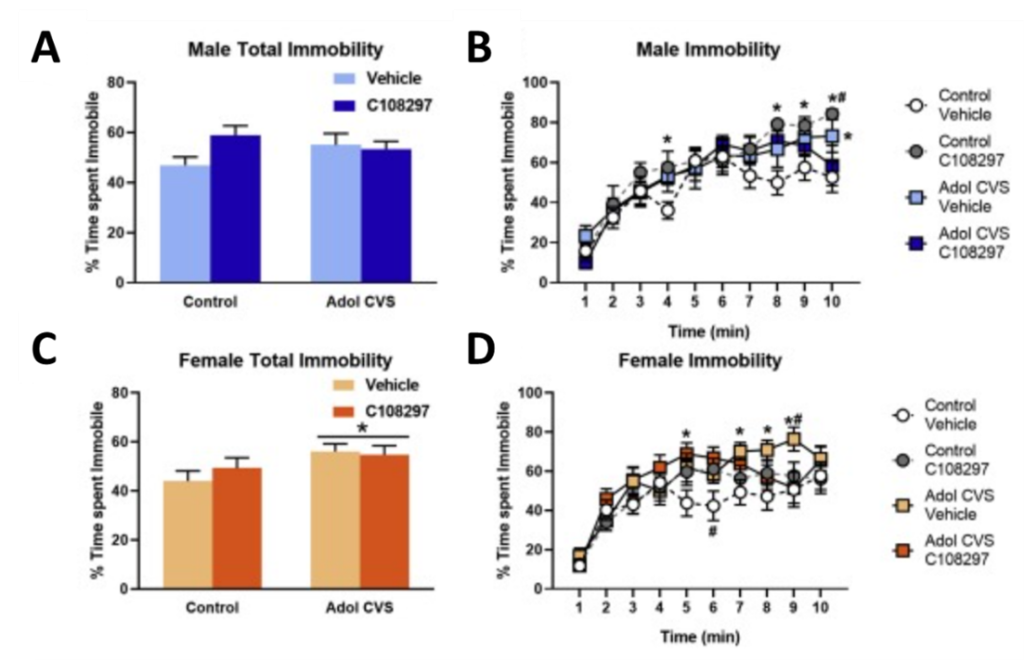
How Does Adolescent Stress Change Physiological Response to a Novel Challenge?
In addition to measuring coping style, the forced swim test allowed researchers to gauge how chronic stress during adolescence may alter physiological response to a new stressful event. Following forced swim tests, blood samples were taken every 15 minutes to analyze for levels of the stress hormone corticosterone, as a measure of stress reactivity. Interestingly, while there was no effect of adolescent stress on circulating levels of corticosterone in males, stressed females had a sharp increase in corticosterone levels lasting 30 minutes after the forced swim test. Importantly, the stressed female group treated with the GR modulator during adolescence did not display this spike in corticosterone, and more closely resembled the control group that was not stressed in adolescence. This outcome indicates that GR modulation may protect against stress-induced HPA axis dysregulation, specifically in females.
A History of Stress May Change the Brain’s Response to New Stressors
Last up on this team of researchers’ impressive to-do list was to analyze neuronal activity in specific brain regions. This was done using a technique called immunohistochemistry, which uses antibodies (like the kind that make up our immune system) that bind to specific proteins in brain tissue, leading to fluorescence that can be detected via microscope (6). Different antibodies are commercially available to detect a wide range of proteins found in the brain. This paper used an antibody that detects the protein Fos, whose production is correlated with neuronal activity (7). So, areas with higher neuronal activity will produce more Fos, which will lead to high levels of fluorescence. Interestingly, researchers found decreased Fos expression after forced swim in the stressed males in areas like the prefrontal cortex (a brain area involved in executive decision making) and the amygdala (involved in emotion processing; see figure below). This means a history of adolescent stress may have dampened the ability of these stress-responsive brain areas to activate in the face of a new stressor in the male rats.
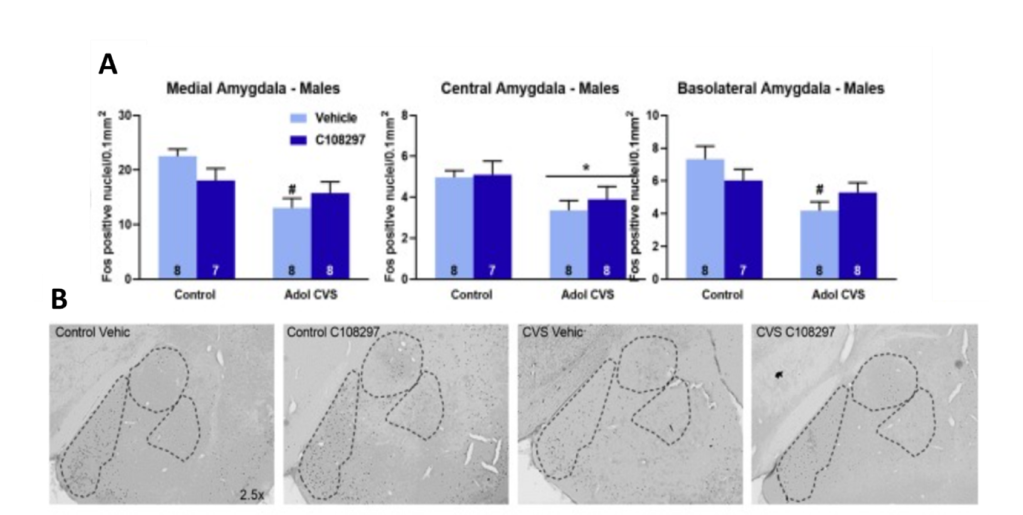
The Sex-Specific Consequences of Adolescent Stress
To summarize, following adolescent stress, adult females became more passive in their coping strategy as measured by time immobile during forced swim. This correlated with elevated corticosterone levels lasting 30 minutes after forced swim, indicating increased stress reactivity. Importantly, these effects may be attenuated by administration of the GR modulator. In contrast, males displayed no such changes in behavior and physiology following adolescent stress. They do, however, show decreased activity in stress-responsive brain areas important for coordinating integration of information and response to stressful stimuli. Researchers propose that the decreased activity in areas of the amygdala and cortex may actually be protective and lead to some of the stress resilience observed in males.
These results indicate how the effects of chronic adolescent stress vary with biological sex. While a history of adolescent stress induces a shift towards passive coping style in females when faced with future stressors, males appear to have some level of pre-existing stress resilience. Although stress in humans is often more varied and complex, resulting in cognitive and emotional consequences that can be challenging to model in rodents, this study provides evidence that males possess some biological advantage promoting stress resiliency. While the complete neural mechanisms mediating this sexually dimorphic response are yet to be uncovered, this research identifies glucocorticoid receptors as a potential target in rescuing some adolescent-stress induced behavioral deficits.
Additional References
- Spencer, R. L., & Deak, T. (2017). A users guide to HPA axis research. Physiology & behavior, 178, 43–65. https://doi.org/10.1016/j.physbeh.2016.11.014
- Gjerstad, J. K., Lightman, S. L., & Spiga, F. (2018). Role of glucocorticoid negative feedback in the regulation of HPA axis pulsatility. Stress (Amsterdam, Netherlands), 21(5), 403–416. https://doi.org/10.1080/10253890.2018.1470238
- Misiak, B., Łoniewski, I., Marlicz, W., Frydecka, D., Szulc, A., Rudzki, L., & Samochowiec, J. (2020). The HPA axis dysregulation in severe mental illness: Can we shift the blame to gut microbiota?. Progress in neuro-psychopharmacology & biological psychiatry, 102, 109951. https://doi.org/10.1016/j.pnpbp.2020.109951
- Slattery, D. A., & Cryan, J. F. (2012). Using the rat forced swim test to assess antidepressant-like activity in rodents. Nature protocols, 7(6), 1009–1014. https://doi.org/10.1038/nprot.2012.044
- Yankelevitch-Yahav, R., Franko, M., Huly, A., & Doron, R. (2015). The forced swim test as a model of depressive-like behavior. JoVE, (97), 52587. https://doi.org/10.3791/52587.
- Magaki, S., Hojat, S. A., Wei, B., So, A., & Yong, W. H. (2019). An Introduction to the Performance of Immunohistochemistry. Methods in molecular biology (Clifton, N.J.), 1897, 289–298. https://doi.org/10.1007/978-1-4939-8935-5_25
- Perrin-Terrin, A. S., Jeton, F., Pichon, A., Frugière, A., Richalet, J. P., Bodineau, L., & Voituron, N. (2016). The c-FOS Protein Immunohistological Detection: A Useful Tool As a Marker of Central Pathways Involved in Specific Physiological Responses In Vivo and Ex Vivo. Journal of visualized experiments : JoVE, (110), 53613. https://doi.org/10.3791/53613
Image Credits
Featured Image- created by CIPHR Connect, under Creative Commons Attribution 2.0 Generic license. https://www.flickr.com/photos/193749286@N04/51418722107/
HPA axis- adapted from Brian M Sweis, under Creative Commons Attribution-Share Alike 3.0 Unported license. https://commons.wikimedia.org/wiki/File:HPA_Axis_Diagram_%28Brian_M_Sweis_2012%29.png
Forced swim test- created by Data Base Center for Life Sciences, under Creative Commons Attribution 4.0 International license. https://commons.wikimedia.org/wiki/File:201407_porsolt_forced_swim_test.png